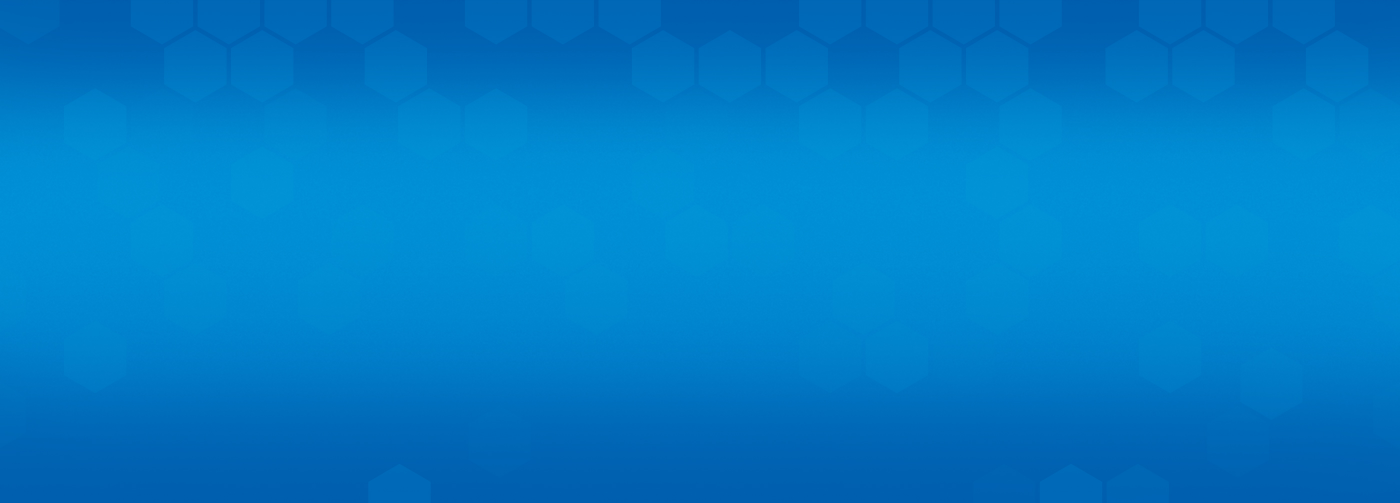
Life Science Optics
In multiphoton microscopy, also known as non linear or two-photon microscopy, a sample is illuminated at twice its normal excitation wavelength. The longer wavelengths are advantageous because they penetrate the sample more deeply for 3D imaging and because they do not damage the sample, allowing increased sample longevity. In order to achieve multiphoton excitation the illumination beam is spatially focused (using optics) and at the same time high-energy short- pulsed excitation beam is used in order to enhance the probability of two (or more) photons arriving at the same location (i.e. fluorophore molecule) at the same time
Examples of multiphoton microscopy techniques include second harmonic generation (SHG), third harmonic generation (THG), coherent anti-Stokes Raman spectroscopy (CARS) and stimulated-emission-depletion (STED) microscopy. Because each of these techniques utilized pulsed lasers, it is important to choose optical components that minimize pulse dispersion, and laser-reflecting dichroics should have low GDD characteristics.
Figure 1: Two-color in-vivo two-photon imaging from the exposed mouse cortex. NADH fluorescence (red) and sulforhodamine-labeled astrocytes (green) taken using BrightLine FF01-680/SP emitter and FF665-Di01 dichroic. Image courtesy of Karl A. Kasischke and Nikhil Mutyal, Dept. of Neurosurgery, University of Rochester Medical Center.
As shown in Figure 2, a typical system is comprised of an excitation laser, scanning and imaging optics, a sensitive detector (usually a photomultiplier tube), and optical filters for separating the fluorescence from the laser (dichroic beamsplitter) and blocking the laser light from reaching the detector (emission filter).
The advantages offered by multiphoton imaging systems include true three-dimensional imaging, the ability to image deep inside of live tissue and the elimination of out-of-plane fluorescence.
With this method it is possible to image fluorescent dyes with very short Stokes shifts and/or very low efficiencies, and even inherently fluorescent molecules native to the sample or tissue. Disadvantages of multiphoton imaging include the need for a high-peak-power pulsed laser, such as a mode-locked Ti:Sapphire laser, and until now, the lack of high-performance optical filters that provide sufficient throughput across the whole emission range of interest and sufficient blocking across the full laser tuning range (Figure 3).
Semrock has brought enhanced performance to multiphoton users by introducing optical filters with ultra-high transmission, very steep edges and carefully optimized blocking. Given how much investment is typically required for the excitation laser and other complex elements of multiphoton imaging systems, these new filters represent a simple and inexpensive upgrade to substantially boost system performance.
The BrightLine® emission filters provide crystal-clear transmission from the near-UV to the near-IR (Figure 4). In fact, the emitters look as clear as window glass in contrast to the brownish tint of traditional filters, and the LWP dichroics have such wide reflection band that they look like highly reflective mirrors (Figure 5). The emission filters also provide deep blocking across the Ti:Sapphire laser tuning range, which is critical to achieving high signal-to-noise ratio and measurement sensitivity.
Sometimes it is desirable to restrict the spectral band of fluorescence emission detected at any given time, especially when multiple fluorophores are used to label different targets in a sample. Narrower bandpass filters are ideal for this purpose, and Semrock provides a wide variety of these bandpass filters that may be combined with a multiphoton emitter with almost no loss of fluorescence signal.
Because nonlinear-optical effects are strongly dependent on the peak intensity of the laser pulse, the most efficient imaging occurs when pulse broadening – and the accompanying decrease in peak intensity – is kept to a minimum. BrightLine FF670-SDi01 and FF720-SDi01 short-wave-pass dichroic beamsplitters are designed to reflect the excitation laser light toward a sample, while offering exceptional transmission of the returning near-UV and visible fluorescence or SHG signal. The group delay dispersion (GDD) associated with laser light reflected off of these beamsplitters is very low. For example, a 100 fs transform-limited Gaussian pulse experiences less than 2% broadening over the full laser wavelength range for the FF670-SDi01 filter, and much less than 1% broadening over the full laser wavelength range for the FF720-SDi01 filter. Learn More about GDD
Figure 2: Typical configuration of a multiphoton fluorescence microscope.
Figure 3: Multiphoton microscopes require control of light over a very wide spectrum: from the near-UV all the through the near-IR.
Figure 4: BrightLine multiphoton filters provide nearly ideal performance, as shown in these typical measured spectra of the "Near-UV & Visible" emitter FF01-680/SP and dichroic FF665-Di01.
Figure 5: BrightLine multiphoton filters are crystal clear!
Figure 6: Research using Semrock multiphoton filters demonstrates the power of fluorescent Ca2+ indicator proteins (FCIPs) for studying Ca2+ dynamics in live cells using two-photon microscopy. Three-dimensional reconstructions of a layer 2/3 neuron expressing a fluorescent protein CerTN-L15. Middle: 3 selected images (each taken at depth marked by respective number on the left and right). Image courtesy of Prof. Dr. Olga Garaschuk of the Institute of Neuroscience at the Technical University of Münich. (Modified from Heim et al., Nat. Methods, 4(2): 127-9, Feb. 2007.)
Optical Filters for Laser-based Fluorescence Microscopes
Optical Filters for Laser-based Fluorescence Microscopes
Read our cover story in BioPhotonics - Optical Filters for Multiphoton Microscopy (Nov 2006)
Nikon Microscopy U - Fundamentals and Applications in Multiphoton Excitation Microscopy