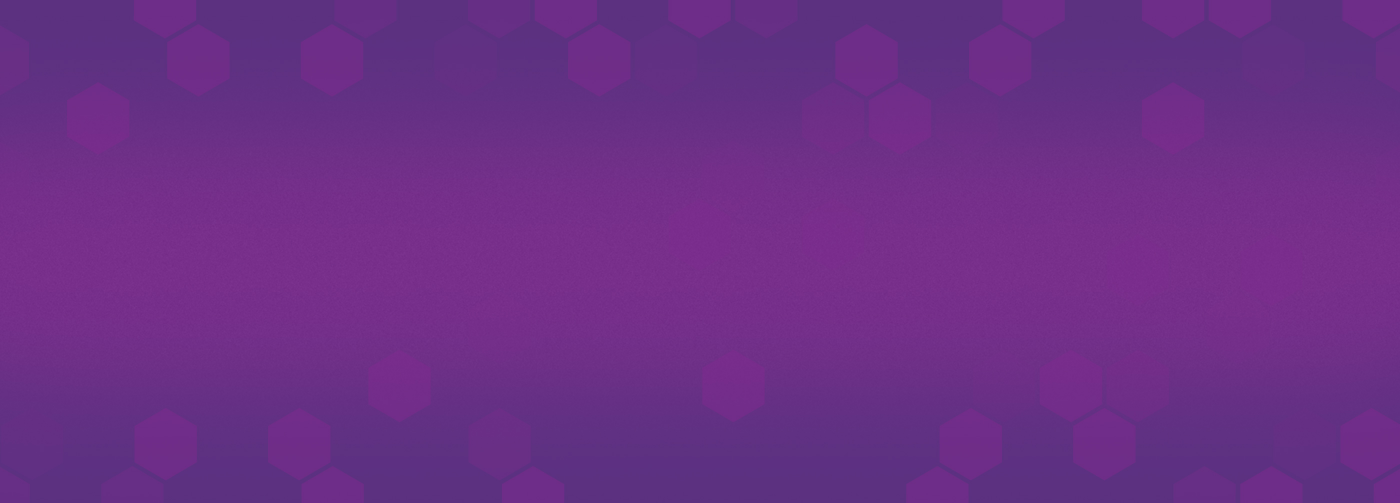
Chris Schaffer is an associate professor in the Meinig School of Biomedical Engineering at Cornell University. He co-runs Cornell’s Schaffer – Nishimura Lab, which develops advanced optical techniques that enable the study of neurological disease through quantitative imaging and manipulation of cells.
IDEX Health & Science: You received your Ph.D. in physics from Harvard, and then completed postdoctoral research in a neuroscience laboratory at UC San Diego. What drew you to neuroscience?
Dr. Schaffer: As I was finishing up my PhD in physics, I had developed some optical tools that I thought would be useful for biology, and I wanted to be one of the people that helped to deploy those optical tools for biomedical research. There was also this sense at the time that there was an increased need for people with quantitative and instrumentation backgrounds to contribute to biological and biomedical research, so there was a real draw.
In terms of why it was neuroscience, that was really just happenstance. My partner, Nozomi Nishimura, and I met when I was a grad student and she was an undergrad at Harvard. She decided to go to UCSD for her PhD, and so when I was looking for post-docs I was looking at UCSD. David Kleinfeld’s lab, where I ended up going, was a hybrid; some of the people in there were from physics, some were from neuroscience, and so it looked like a good lab to transition from doing straight physics work to doing more applied physics and biomedical research. I can’t say that I was strongly drawn toward neuroscience; I was looking for applications in biology and this was the opportunity where I was able to do that.
IDEX Health & Science: What inspired you to start your own lab at Cornell?
Dr. Schaffer: Since I was a college student I’ve had this idea that I wanted to be an academic. I think I probably could have been an academic in just about any discipline, but in terms of my interests I think I’m drawn primarily to the lifestyle and the idea of being a scholar. I did well in physics as an undergraduate and in graduate school, so I just sort of built from there. But I’ve long wanted to run my own lab.
IDEX Health & Science: In your current work at the Cornell lab, you use advanced optical techniques not only to see what’s going on in the brain, but also manipulate it in order to investigate diseases. Can you tell me more about that?
Dr. Schaffer: We’ve developed what you can think of as a laser scalpel that can cut with micron-scale precision down inside tissue without affecting the things above it. There is some potential for therapeutic and surgical applications of this, and in fact, we weren’t the only ones that seized on this idea and developed it. One of the places where it’s been most successful is in the surgical treatment of myopia. You’ve probably heard of Lasik surgery, where the cornea is reshaped in order to make your eye focus sharply on the retina. There’s a step in that procedure where the same kind of laser scalpel that we use for biomedical research is used to help facilitate that corneal reshaping.
We use it for a few different things. For example, we just had a paper come out, with Joe Fetcho here at Cornell University, where we used that scalpel to kill individual neurons or even to cut individual dendrites or axons off of neurons in the brain of a zebrafish. We’re actually changing the wiring of the neural network in the zebrafish. And then we looked at how that affected the electrophysiological properties of the network and how that affected the behavior of the animal. That’s an example of using that laser scalpel to perturb a biological system in a very precise way, and then look at how that changes the system in order to understand the function of the thing that you perturbed.
Image of blood vessels on the surface of the brain of a live rat. Image courtesy Schaffer – Nishimura Lab.
We also use it to model disease states. In my postdoc at UC San Diego in David Kleinfeld’s laboratory, we actually developed the use of that technology to injure small blood vessels in the brains of rodents; this gave the first useful animal model of what you would call a micro-hemorrhage, where a small blood vessel bleeds. The more of these little bleeds you have, the worse you are off cognitively. With this laser scalpel we can just knick the tiny blood vessel— arteriole, capillary, or venule—down in the brain. It causes a little bit of bleeding, and then through in vivo imaging, histopathology, a variety of techniques, we can study how that small bleeding affects the health and function of nearby brain cells. The goal is then to identify mechanisms that you can try to block or enhance in order to provide therapeutic options for people who have lots of these little bleeds. We and others have done similar work where we used this laser scalpel not to cause a blood vessel to rupture but just to injure the inside of it enough that it triggers the clotting cascade that leads to the formation of an occlusion.
So it’s a physical manipulation tool that you can use to modify the structure of some biological systems in a very precise way for studying function, or try to induce a problem in a biological system to try to model a disease state.
IDEX Health & Science: One of your major breakthroughs has been in understanding how interruptions in blood flow to the brain contribute to Alzheimer's disease. Can you talk about the research and techniques that lead to this discovery?
Dr. Schaffer: The work we have in Alzheimer’s disease doesn’t make use of that laser scalpel, but it does make use of advanced optical imaging techniques, like two-photon-excited fluorescence microscopy, to be able to image down deep into the brain of mice to study the physiology of what’s going on. Two-photon-excited fluorescence microscopy is an imaging technique that allows you to map the 3-dimensional distribution of fluorescently labeled objects with micron-scale resolution down deep into scattering tissues like the brain. We can image a millimeter down into the brain of a mouse, which is basically the thickness of the cortex.
What we were trying to understand is why patients with Alzheimer’s disease have reduced blood flow to their brain. There’s about a 30% reduction in blood flow in the brain of patients who have Alzheimer’s as compared to those who don’t. And that much blood flow reduction contributes to cognitive symptoms; it’s an important part of the disease. People have known about this for a long time, but the mechanism that leads to that reduced blood flow is unclear. So we use high resolution imaging of blood vessels, and we can actually measure whether or not those blood vessels are flowing or if they have an obstruction. What we found was that in Alzheimer mice, while the larger blood vessels in the brain didn’t show any signs of clotting or obstruction, when we looked at the tiniest blood vessels, individual brain capillaries, we found that about 2% of capillaries have a cell that plugs blood flow in them in Alzheimer’s disease mice, while in wild type or normal mice, basically all of the capillaries are flowing. This suggested to us that maybe the problem in blood flow is at the capillary level. We recently identified what kind of cell it is, and some of the molecular interactions that are playing a role, so we’re able to block these interactions and cause the stalled capillaries to start flowing again, leading to a ~30% increase in overall brain blood flow. Accompanying that increase in blood flow is a dramatic improvement in multiple different measures of cognition, such as spatial and working memory and measures of depression. And there’s even some slowdown in the accumulation of some species of amyloid beta, the protein that aggregates to drive development of Alzheimer’s disease.
Using high resolution imaging to understand the cellular interactions that cause specific symptoms of the disease, in this case reduced brain blood flow, has given us hints about a new pathway that could be important for Alzheimer’s therapy.
IDEX Health & Science: You’ve just launched an innovative game that allows the public to take part in your Alzheimer’s research. Tell us about this project.
Dr. Schaffer: Stall Catchers is a science game that tasks individuals with looking at movies of real blood vessels in the mouse brains we’re studying, and searching for areas where blood is no longer flowing. You don’t have to have any sort of background in science to play; the game gives you instructions. Players can level up and receive digital badges for their achievements, and at the same time they’re contributing data that will help us answer important questions about the disease.
The game was developed by the Human Computation Institute as part of EyesOnALZ’s Citizen Science Project. It uses the platform created by Andrew Westphal of Berkeley for stardust@home, a game that lets players search for tracks of stardust in layers of aerogel. Andrew himself did a lot of the work to get Stall Catchers going, and we’re very grateful for his efforts.
IDEX Health & Science: What would you consider your most significant achievement to date?
Dr. Schaffer: I’m always most excited about what we’re doing now. This work on understanding the underlying mechanisms for decreased brain blood flow in Alzheimer’s disease, we’re very excited about. If this also turns out to be the mechanism in humans, then improving brain blood flow in Alzheimer’s patients could really change the course of that disease for a lot of people. At this point it’s just initial data in mice, so it’s a long way before we get there, but it’s very interesting and we’re very excited about it.
IDEX Health & Science: What is the next disease or challenge you hope to tackle?
Dr. Schaffer: I run my lab jointly with my partner, Nozomi Nishimura, and between the two of us we have a broad range of projects. Right now we’re trying to image patterns of neural activity in the brain and the spinal cord of mice while they’re walking, in order to understand how animals coordinate their locomotion. How do you walk, and then increase your pace, and then slow back down, all without your brain having to tell you right leg, left leg, right leg, left leg? You’d never get anywhere if you had to do that. All of that stuff is controlled at the level of the spinal cord; exactly how it works isn’t totally clear, although there’s a lot of good data already out there. We’ve developed a suite of imaging tools that let us image patterns and neural activity in the spinal cord in mice while they’re walking, and I hope we’ll be able to uncover how those underlying neural circuits work. We are pursuing this project in part because it’s just interesting neuroscience, trying to understand how your brain does a fundamental task that all land animals have to do. Then also it will be interesting to understand how those things are altered by mild spinal cord injuries, neurodegenerative diseases like ALS, and other things that affect movement and locomotion. We’re just getting the tools and techniques together right now, but I hope that it’ll have a good future.
IDEX Health & Science: How have Semrock products helped you in your work?
Dr. Schaffer: Two-photon excited fluorescence microscopy relies on the capability to separate fluorescent light by its color with different fluorescent molecules tagged to different kinds of cells, so we can study cellular interactions. It’s essential for us to have high quality optics that we can use to separate emitted fluorescence or to combine different laser beams, in order to be able to get as much information as we can from microscopy. We’ve used Semrock as a supplier for those kinds of dichroic and interference filters for many years.
Semrock has also been a partner for us on one of our projects aimed at developing new kinds of microscopy. About a third of what we do in the lab is what you could think of as applied physics; we’re trying to develop new optical tools and techniques for biomedical research. One of the tools that we’re trying to develop is something called a hyperspectral multiphoton microscope. In a normal multiphoton fluorescence microscope you might image two different wavelength bands, and with that you could label two different kinds of cells and see how they interact with each other, but you’d be blind to all the other cells that are around and what they might be doing. Our goal is to get to the point where we’re not blind like that, where we basically have every single cell in an image volume fluorescently labeled in a way where we can distinguish them by cell type, or even distinguish individual cells within a type from each other. There are fluorescent labeling strategies now that would enable us to do that, but the problem is that then you would have to separate lots and lots of different fluorescent markers whose emission colors and excitation profiles are not that different from each other. So in order to separate those cleanly, we really need to be able to take lots and lots and lots of color data, or what people would call hyperspectral data.
Multiphoton Hyperspectral Microscopy utilizing VersaChrome® Tunable Filters. Composite of ten bead colors embedded in agarose (A), a live, dividing cell with four fluorescent labels (B; blue – Endoplasmic Reticulum, green – Plasma Membrane, red – Nucleus, yellow – Microtubule Ends), and live mouse cortex labeled with three fluorescent proteins delivered through AAV2 viral vector (C). Six fluorescent labels are cleanly unmixed from an image of live mouse cortex (D; from left to right, nuclei, microglia, vasculature, neuron dendrites, and astrocytes). The final composite shows all cell types simultaneously (E; blue - nuclei, green – microglia, cyan – vasculature, yellow – neurons, red – astrocytes). Image courtesy Schaffer – Nishimura Lab.
Semrock a few years ago came out with their VersaChrome filters; these are interference filters where as you change the incidence angle of the light on them, the color of light they transmit shifts, while maintaining a high transmission. When we learned about these filters we actually designed a microscope around them. This microscope takes about 50 channels of excitation/emission color information per pixel, so it’s like a 50-color microscope. We’re now able to have a dozen different dyes or fluorescent molecules in the sample at the same time, and we’re able to distinguish those colors from each other very accurately. That will allow us to image the dynamics not just of a couple of cells as they interact but many different kinds of cells, and eventually all cells in a tissue volume. Semrock has been a key contributor to helping us develop some of these new microscopy techniques in biomedical research.
IDEX Health & Science: What’s your take on the optics industry overall and how it’s advancing?
Dr. Schaffer: Optical technology across the board has been a dramatically expanding industry since I got into it. Much of that has been in telecommunications and things like that. But in biomedical research, and specifically in neuroscience, I think optical technologies are coming to dominate, really. The way biological systems work is by the coordinated activity of lots of the same and lots of different kinds of cells that are arranged in some spatial pattern and interacting with each other over time. So it turns out that optics is just a good way to study that. Thanks to high spatial resolution, we can visualize individual cells and sub-cellular features, and look in live animals without damaging cells. With fluorescent labeling strategies we can distinguish different kinds of cells or even quantify cell function; for example, there are molecules whose fluorescence intensity will change when a neuron fires an action potential, so that allows neural activity patterns to be recorded with light. Optics is a really powerful tool that has applications across many different fields of biomedical research.
Laser amplifier crystal providing high-power, femtosecond duration pulses. Image courtesy Schaffer – Nishimura Lab.
I see the use of optical tools in biomedical research only growing over time. In particular, this imaging technique, two-photon excited fluorescence microscopy—the first paper on it was actually published from here at Cornell in the early 1990s, and this is now the dominant in vivo imaging technique around the world. Over the last 30 years this has gone from something that nobody did to something that is a commonplace technique.
IDEX Health & Science: You spent some time as a science policy advisor for U.S. Senator Ed Markey. Can you tell us a bit about that and how it has influenced your work since then?
Dr. Schaffer: I wouldn’t say it’s influenced my scientific work, specifically. I’ve long had an interest in policy and politics, and when my first sabbatical came up, I applied for one of the American Association for the Advancement of Science Congressional Science & Technology Policy Fellowships, and won one. My fellowship was funded by SPIE and the Optical Society of America. The goal of that program is basically to have PhD level scientists spend a year or two working in the federal government, trying to do a couple of things: One is to bring quantitative approaches to analyzing policy decisions and bring real science to bear on making policy decisions, both in Congress as well as in the various branches of the administration. A second goal is to improve the policies that are made around the conduct of science: regulatory policy around science, funding, things like that. It is a little bit like throwing you in the deep end and seeing how well you swim.
I spent a year in Senator Markey’s office. I worked on a broad range of issues: safety in nuclear power reactors for electricity generation, U.S. nuclear weapons portfolio, cyber security, science education and research funding. I also worked on some things that weren’t quite as directly science related; I worked on some airline security issues, a little bit of foreign policy stuff. For me it was a wonderful experience. It was very interesting to learn how policy is made and the kinds of information that is used and the processes that things go through before policy is formulated. I gained a great deal of respect for our system of government and for the people who work in it, both those who are aligned with me politically as well as those who have different policy views.
And I’ve remained very active in policy. I’m down in D.C. quite a bit now advocating for increased use of scientific data and ideas in formulating policy, and improved policies for the conduct of science. I also run a training program at Cornell that aims to help graduate students and post-docs who are interested in policy applications figure out whether or not it’s what they want to do and then, if it is, to gain some of the experience and credibility that would help them secure a position doing that kind of work.
IDEX Health & Science: You’ve also been involved in developing strategies for science education at the middle and high school level. What advice would you give young people interested in pursuing a career in optics or science in general?
Dr. Schaffer: Certainly if your goal is a career in optics or in science, I think becoming actively involved in science as soon as you can is important. These days, through the K-12 level and frankly even at the undergraduate level, we mostly teach science as a collection of static facts in a book. And that’s not what science is. That’s kind of like science history or science reference, and I don’t want to discount that—you do need to know a lot of things in order to be a successful scientist. But science is much more about the creative process for discovery. It’s about getting up to the point where you are at the boundary of human knowledge, and you’re trying to develop ideas and test them and do discovery-driven experiments to try to extend that boundary. And that feels very, very, very different than learning about the science that’s already been done.
I think it’s important to understand what it’s like to be a professional scientist before you decide that you’re going to do it for your career, and the only way you’re going to get a feel for what that’s like is by doing original scientific research. There are lots of opportunities to do this as an undergraduate and even some earlier. In my own lab we have between 20 and 25 undergraduate researchers right now; they work in the lab for years and publish as authors on papers, and really get a feel for what it’s like to work as a professional scientist. This past summer we had four high school students who were doing internships. So for people who think their goal might be to become a scientist and a researcher, I’m not saying you shouldn’t take science classes and read science books, because you definitely have to build up your knowledge base—but don’t just to that. Become involved as early as you can in a project that involves new, novel scientific research.